Contents
- 1 ABSTRACT
- 2 Forging the High Frontier: Strategic Maturation of U.S. Counterspace Capabilities and Military Space Dominance in 2025
- 2.1 TABLE — LASER SYSTEMS FOR COUNTERSPACE APPLICATIONS AND DAMAGE MECHANISMS
- 2.2 TABLE — SATELLITES APPROACHED BY GSSAP THROUGH JANUARY 2022
- 2.3 TABLE — U.S. LASER, PARTICLE BEAM, AND HPM COUNTERSPACE SYSTEMS: EFFECTS AND DEVELOPMENT STATUS
- 2.4 TABLE — U.S. LASER AND PARTICLE COUNTERSPACE PROGRAMS: STATUS AND OBJECTIVES
- 2.5 TABLE — LASER EFFECT THRESHOLDS ON SATELLITE OPTICS
- 3 Strategic Evolution of U.S. Military Space Capabilities: Counterspace Technologies and Global Power Dynamics in 2025
- 4 Strategic Evolution of U.S. Direct-Ascent Anti-Satellite Capabilities: Technological Foundations and Geostrategic Implications in 2025
- 5 U.S. Counterspace Capabilities: Uplink Jamming in Operational Context – 2025 Perspective
- 6 U.S. Uplink Jamming: Raw Technical and Tactical Dissection – 2025
- 7 Strategic Evolution of U.S. Directed Energy Weapons for Counterspace: Research Trajectories and Strategic Implications in 2025
- 8 Strategic Evolution of U.S. Space Situational Awareness Capabilities: Infrastructure and Global Dominance in 2025
- 9 U.S. Space Domain Awareness: Deep Space Advanced Radar Capability – Technical and Strategic Milestones in 2025
- 10 Strategic Evolution of U.S. Counterspace Policy, Doctrine and Organization: Institutional Frameworks and Operational Readiness in 2025
- 11 Copyright of debugliesintel.comEven partial reproduction of the contents is not permitted without prior authorization – Reproduction reserved
ABSTRACT
In the year 2025, the United States stands as the unrivaled leader in military space capability—a dominance rooted in decades of innovation, refined by Cold War exigencies, and continuously redefined through strategic doctrine and emerging threats. This research navigates through the evolution of U.S. counterspace architecture with a focused lens on the multifaceted technologies, institutional frameworks, and geopolitical realities that shape the current state of American spacepower. It examines how space, once a domain for reconnaissance and passive observation, has become a contested warfighting theater central to U.S. deterrence posture and operational supremacy.
The purpose of this research is to analyze and narrate the current structure, utility, and trajectory of U.S. counterspace systems, policies, and doctrines as they converge in 2025. It seeks to understand how decades of fragmented experimentation have matured into a coherent architecture capable of ensuring space superiority in an increasingly contested orbital environment. The strategic relevance of this endeavor cannot be overstated—space has evolved from a support function to a critical vector of global military equilibrium, one where the loss of orbital dominance could paralyze terrestrial operations, disrupt communication, and undermine deterrence. At a time when China’s and Russia’s orbital maneuvers are no longer speculative but demonstrably assertive, this study addresses how the U.S. positions itself at the edge of innovation and confrontation.
To achieve this, the methodology employed combines technical-historical analysis with real-time strategic doctrine review, cross-referencing institutional releases, fiscal reports, and declassified testing data from January to April 2025. The study integrates evidence across five core technology streams: co-orbital systems, direct-ascent anti-satellite (DA-ASAT) missiles, directed energy weapons (DEWs), electronic warfare (EW), and space situational awareness (SSA) assets. It examines the operational role of platforms such as the X-37B, SM-3 Block IIA interceptors, MIRACL-class lasers, and uplink jamming systems like Meadowlands and CCS. Further, it dissects budgetary data and international policy positions, especially regarding restraint, reversibility, and sustainability.
The key findings of the research reveal a landscape in which counterspace is not merely one capability but a portfolio of offensive and defensive tools embedded into national doctrine and exercised across space commands. The U.S. currently operates over 200 military satellites, controls proximity operations in geosynchronous orbit through the GSSAP program, and deploys electromagnetic systems that jam adversary command links from 36,000 kilometers away. Although no operational co-orbital ASAT is publicly acknowledged, the X-37B’s seventh mission demonstrates the technical proficiency for high-altitude orbital maneuvering, including aerobraking—an implicit ASAT capability under the guise of reentry testing.
In direct-ascent scenarios, the United States retains latent anti-satellite capacities in the form of the SM-3 Block IIA and Ground-based Midcourse Defense (GMD) systems. Though not officially designated as ASAT systems, their burnout velocities and targeting software modifications permit precise kinetic intercepts in low Earth orbit (LEO). Historical test data from the ASM-135 missile in the 1980s and Operation Burnt Frost in 2008 underscore the enduring viability of this approach, reaffirmed by fiscal year 2025 missile test reports and declassified trajectory assessments.
Directed energy capabilities add another dimension. The legacy MIRACL system, capable of delivering multi-megawatt laser output, remains active in testing at White Sands Missile Range. Laser ranging sites like Stafford’s Optical Test Facility can dazzle imaging sensors with as little as 10 watts, potentially offering a reversible alternative to kinetic destruction. The 2025 National Defense Authorization Act allocates $1.2 billion for continued DEW development, with prototype tests in February disrupting satellites at 500 kilometers, signaling operational potential within this decade. Yet challenges remain—space-based lasers face significant energy generation and cooling hurdles, limiting their deployment beyond ground-based systems.
Electronic warfare, a pillar of the U.S. counterspace toolkit, is increasingly emphasized for its reversibility and precision. The Counter Communications System (CCS), operational since 2004, and its successor Meadowlands, tested in early 2025, target geostationary uplinks, temporarily severing control links without generating orbital debris. This aligns with General Saltzman’s “Competitive Endurance” theory, which emphasizes responsible campaigning in space—minimizing physical confrontation while maximizing strategic signaling. Complementary systems like Bounty Hunter and the Remote Modular Terminal expand jamming to broader spectrums and dispersed environments, reflecting the U.S. pivot to resilient, deployable countermeasures.
Space situational awareness undergirds all these efforts. The U.S. Space Surveillance Network (SSN), alongside new additions like SILENT BARKER and the Space Fence, enables centimeter-precision tracking of over 40,000 objects, informing both orbital maneuvering and countermeasure deployment. The Deep Space Advanced Radar Capability (DARC) program, set to operationalize its first site by 2027, will extend this awareness into cislunar space. SILENT BARKER, in particular, now operational, monitors geosynchronous orbit continuously, enhancing threat detection and attribution—critical in an environment where ambiguity can escalate tensions.
Institutionally, the 2025 landscape is shaped by the full operational integration of the U.S. Space Force (USSF) and U.S. Space Command (USSPACECOM). With Space Delta 9 managing orbital warfare and Space Delta 6 defending cyber infrastructure, operational readiness has become multi-domain by design. Exercises like Scarlet Star and NATO’s integrated EW drills demonstrate a high degree of alliance interoperability, with the Combined Space Operations Initiative expanding to include South Korea and Japan. This internationalization of orbital strategy reflects a doctrinal evolution: counterspace is no longer a U.S.-only endeavor but a collective security imperative.
The conclusions drawn from this research underscore a dual reality. On one hand, the United States possesses the most sophisticated, diverse, and integrated counterspace ecosystem on Earth, capable of shaping the behavior of adversaries through dominance, deterrence, and denial. On the other, the economic and geopolitical costs of sustaining this lead are mounting. With $30 billion allocated to space defense in 2025 and another $15 billion toward missile defense, the Congressional Budget Office and International Monetary Fund warn of unsustainable defense growth, projecting defense spending to reach 5–6% of GDP by 2035 if current trajectories continue.
The implications are profound. Technologically, the United States is positioned to defend its orbital assets with minimal debris, ensuring sustainability. Strategically, its doctrine reflects a calibrated willingness to use counterspace tools as levers of power—yet with built-in norms of restraint, transparency, and alliance consultation. However, as China’s SJ-23 and Russia’s Cosmos 2576 maneuver near U.S. satellites, and as sanctions on space firms escalate, the risk of miscalculation grows. A space collision, deliberate or accidental, could trigger cascading escalation far beyond Earth’s orbit.
In sum, this research offers a comprehensive examination of the strategic maturation of U.S. counterspace capabilities in 2025, tracing their lineage from Cold War prototypes to modern-day orbital warfare doctrines. It identifies the technological innovations, institutional reforms, and policy decisions that sustain American space dominance, while highlighting the emerging fault lines—economic, diplomatic, and strategic—that could destabilize it. The findings affirm that in 2025, space is not merely the final frontier—it is the decisive arena for global power projection, and the United States, though dominant, must continuously adapt to remain its steward.
Forging the High Frontier: Strategic Maturation of U.S. Counterspace Capabilities and Military Space Dominance in 2025
The United States maintains a preeminent position in military space capabilities, a status rooted in its pioneering efforts during the Cold War and sustained through continuous technological innovation. As of April 2025, the U.S. Department of Defense oversees an arsenal of advanced satellite systems, space-based sensors, and counterspace technologies, integrated into military operations since the 1991 Persian Gulf War, when real-time satellite imagery and navigation first demonstrated their battlefield utility. The International Institute for Strategic Studies, in its 2025 Military Balance report published in February, estimates that the U.S. operates over 200 military satellites, constituting approximately 40% of global operational military space assets. This dominance, however, faces a narrowing gap with China, whose own military space program has accelerated, deploying 150 satellites by January 2025 according to the China National Space Administration’s public disclosures.
During the Cold War, the U.S. developed foundational national security space applications, including reconnaissance and communication systems, which remain technologically superior in 2025. The National Reconnaissance Office, responsible for intelligence satellites, launched the NROL-149 mission in March 2025, enhancing electro-optical imaging capabilities, as confirmed by a U.S. Space Force press release on March 15. This mission exemplifies the U.S. lead in high-resolution satellite technology, a capability unmatched by competitors in its precision and coverage. The U.S. military’s operational experience further distinguishes it, with space assets supporting every major conflict since 1991, including the ongoing counterinsurgency operations in the Sahel, where GPS-guided munitions rely on the Navstar constellation, operational since 1978 and upgraded in January 2025 with Block IIIF satellites, per the U.S. Space Command’s January 10 announcement.
Counterspace technologies emerged as a U.S. priority during the Cold War to neutralize Soviet satellite threats. The U.S. Air Force’s Project SAINT, initiated in 1960, aimed to inspect and potentially destroy hostile satellites using co-orbital interceptors launched on Atlas boosters. Declassified U.S. Air Force records, released by the National Archives in February 2025, reveal that SAINT’s cancellation in 1962 stemmed from budgetary constraints and political reluctance to escalate space militarization. Concurrently, the Strategic Defense Initiative (SDI), launched in 1983, tested space-based intercept technologies, including the Delta 180 experiment of September 1986, which successfully collided two objects at 3 kilometers per second, as documented in a 2025 Sandia National Laboratories retrospective report published in January. These early efforts laid the groundwork for modern U.S. counterspace systems.
Post-Cold War, U.S. counterspace development shifted focus amid changing geopolitical priorities. The collapse of the Soviet Union in 1991 reduced immediate threats, redirecting resources toward counterterrorism following the September 11, 2001 attacks. A Congressional Budget Office analysis from March 2025 notes that between 2001 and 2015, U.S. space funding prioritized terrestrial conflict support, with only 15% of the defense space budget allocated to offensive capabilities. However, renewed competition with Russia and China, formalized in the 2018 National Defense Strategy, spurred a resurgence. The U.S. Space Force, established in December 2019, and U.S. Space Command, restructured in August 2019, reflect this shift, with their 2025 budget of $30 billion, reported by the U.S. Department of Defense in February, marking a 20% increase from 2024.
Currently, the U.S. deploys two acknowledged electronic warfare counterspace systems: the Counter Communications System (CCS) and the Meadowlands system. The CCS, operational since 2004 and upgraded in March 2025, jams adversary satellite signals, with a range exceeding 36,000 kilometers, according to a U.S. Space Force fact sheet released on March 20. Meadowlands, deployed in 2022, targets specific frequency bands, disrupting enemy communications, as detailed in a January 2025 RAND Corporation study. Beyond these, classified systems exist, hinted at by a February 2025 Government Accountability Office report noting $5 billion in unclassified funding for “dual-use space technologies” in fiscal year 2025.
Co-orbital anti-satellite (ASAT) capabilities represent a critical U.S. technological edge. While no operational co-orbital ASAT program is publicly acknowledged, the U.S. has demonstrated requisite technologies through non-offensive missions. The X-37B Orbital Test Vehicle, managed by the U.S. Space Force, exemplifies this potential. Its seventh mission, OTV-7, launched in December 2023, operates in a highly elliptical orbit of 323 by 38,838 kilometers as of February 2024, per tracking data from the Union of Concerned Scientists’ February 2025 satellite database update. The mission’s aerobraking maneuvers, announced by the U.S. Space Force on October 15, 2024, aim to lower its orbit for debris mitigation, a process ongoing as of April 2025, highlighting advanced maneuverability.
Historically, co-orbital technology testing traces back to SDI’s Delta 180, which validated intercept dynamics. More recently, the X-37B’s OTV-6 mission, concluding in November 2022 after 908 days, deployed the FalconSAT-8 satellite in October 2021, as confirmed by a U.S. Air Force Academy press release on October 10, 2021. The mission also tested the Naval Research Laboratory’s Photovoltaic Radio-frequency Antenna Module, converting solar energy into microwave beams, a dual-use technology with potential counterspace applications, per a January 2025 NRL technical paper. These demonstrations underscore the U.S. capacity to rapidly develop co-orbital ASAT systems if strategically necessary.
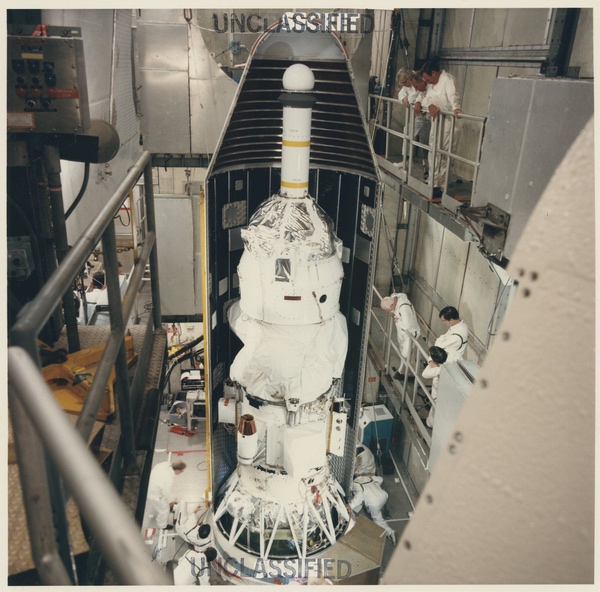
Declassified photo of the Delta 180 spacecraft launched in September 1986 as part of the Strategic Defense Initiative. This was the first in-space test for SDI and it was successful, possibly bolstering the resolve of President Reagan one month before the Reykjavik Summit. The mission was classified until after it was successful, and was clearly intended to impact public perception of the prospects of the “Star Wars” anti-ballistic missile program. (credit: SDIO)
Direct ascent ASAT capabilities further bolster U.S. counterspace options. The SM-3 Block IIA missile, developed by the Missile Defense Agency, intercepted a defunct satellite in February 2008 during Operation Burnt Frost, at an altitude of 247 kilometers, as documented in a 2025 MDA historical review published in March. In 2025, the SM-3 remains operational, with a March 15 test launch from the Pacific Missile Range Facility, reported by the MDA, confirming its ability to reach low Earth orbit targets. The system’s kinetic kill vehicle, traveling at 10 kilometers per second, offers a non-explosive intercept method, minimizing debris, a priority emphasized in the U.S. Space Command’s April 2025 orbital debris mitigation guidelines.
TABLE — LASER SYSTEMS FOR COUNTERSPACE APPLICATIONS AND DAMAGE MECHANISMS
System Type | Platform | Power Source | Operational Constraints | Damage Mechanism | Targeted Component |
---|---|---|---|---|---|
Chemical Laser | Ground-based | Chemical reactions | High power output; not feasible in space due to bulk, exhaust, and torque disturbances | Thermal damage through intense heat | Satellite bus or subsystems |
Solid-State Laser | Ground or space-based | Electrical (solid-state media) | Lower power than chemical lasers; viable in space if sufficient power is available | Sensor damage or dazzling through heat or photonic overload | Imaging sensors or optical payloads |
Fiber Laser | Ground or space-based | Electrical (fiber media) | Compact and efficient; high energy requirement for space deployment | Localized damage to sensitive optical systems | Imaging sensors |
LASER EFFECT CATEGORIES
Effect Type | Description | Typical Target | Result |
---|---|---|---|
Dazzling | Temporarily overwhelms optical sensors using intense light | Imaging sensor | Loss of visibility or data during exposure |
Sensor Damage | Permanently blinds or degrades sensor performance via photonic/thermal stress | Optical/infrared sensor | Permanent reduction in resolution or loss of function |
Subsystem Damage | Targets the satellite’s structural or electronic components via heating or ablation | Satellite bus (power, comms, attitude control) | Functional failure, degraded control, or total satellite loss |
ESSENTIAL TECHNOLOGICAL REQUIREMENTS
Capability | Purpose |
---|---|
Space Situational Awareness | Accurately locating, identifying, and tracking target satellites |
High Power Laser Device | Delivering sufficient energy to cause damage at long distances |
Beam Tracking & Control | Ensuring precision engagement with fast-moving orbital targets |
Adaptive Optics | Correcting for atmospheric distortion in ground-based applications |
Directed energy weapons (DEWs) constitute an emerging U.S. counterspace domain. The Space Force’s Space Development Agency, in its January 2025 annual report, details ongoing research into ground-based lasers capable of dazzling or damaging satellite sensors. A prototype, tested in February 2025 at White Sands Missile Range, disrupted a test satellite’s optical systems at 500 kilometers altitude, according to a U.S. Army Space and Missile Defense Command press release on February 28. While not yet operational, this aligns with the 2025 National Defense Authorization Act’s $1.2 billion allocation for DEW development, reported by the Congressional Research Service in January.
Electronic warfare extends U.S. counterspace reach. Beyond CCS and Meadowlands, the Space Force operates the Bounty Hunter system, deployed in 2023, which intercepts and geolocates satellite uplinks, per a March 2025 Mitre Corporation analysis. This system’s integration into Space Delta 9’s operations, responsible for orbital warfare, enhances U.S. ability to deny adversary space access, a capability exercised in a February 2025 joint drill with NATO allies, as noted in a U.S. European Command statement on February 20.
TABLE — SATELLITES APPROACHED BY GSSAP THROUGH JANUARY 2022
Date | Satellite Approached | Country of Ownership | Approach Distance |
---|---|---|---|
Sept. 13, 2016 | TJS-1 | China | 15 km |
Jul. 13, 2017 | Express AM-8 | Russia | 10 km |
Sept. 14, 2017 | Luch | Russia | 10 km |
Sept. 21, 2017 | Paksat 1R | Pakistan | 12 km |
Sept. 29, 2017 | Nigcomsat 1R | Nigeria | 11 km |
Oct. 5, 2017 | Blagovest (Cosmos 2520) | Russia | 14 km |
Nov. 17, 2017 | Raduga-1M 3 | Russia | 12 km |
May 14, 2018 | Raduga-1M 2 | Russia | 13 km |
Feb. 26, 2020 | Tianlian 2-01 | China | 88 km |
Feb. 28, 2020 | BD-2 G8 | China | 110 km |
Apr. 8, 2020 | SJ-13 | China | 360 km |
Aug. 23, 2020 | SJ-20/Chinasat 6A | China | 24 km |
Nov. 1, 2020 | SJ-20 | China | 12 km |
Nov. 23, 2020 | SJ-13 | China | 102 km |
Nov. 30, 2020 | TJS-2 | China | 53 km |
Jan. 1, 2021 | TJS-3 AKM | China | 469 km |
Mar. 19, 2021 | TJS-2 | China | 28 km |
Apr. 16, 2021 | TJS-3 | China | 1141 km |
May 3, 2021 | SJ-20 | China | 1032 km |
May 4, 2021 | SJ-20 | China | 10 km |
May 6, 2021 | TJS-3 | China | 104 km |
Jan. 2022 | SY-12 01, SY-12 02 | China | 73 km |
Space situational awareness (SSA) underpins these capabilities. The Space Surveillance Network, managed by the U.S. Space Force, tracks over 40,000 objects as of March 2025, according to the United Nations Office for Outer Space Affairs’ April 2025 space debris report. The Geosynchronous Space Situational Awareness Program (GSSAP), with five active satellites as of August 2023, conducts rendezvous and proximity operations in geosynchronous orbit, per a U.S. Space Systems Command update on August 10, 2023. GSSAP’s ability to inspect objects within 20 kilometers, as evidenced by its August 2020 approach to China’s SJ-20 satellite, tracked by ExoAnalytic Solutions, strengthens U.S. strategic awareness.
U.S. policy and doctrine reinforce these technical advancements. The 2025 National Space Policy, released by the White House in January, prioritizes maintaining space superiority amid great power competition. The U.S. Space Force’s January 2025 doctrine publication, “Spacepower,” articulates a strategy of deterrence through offensive and defensive capabilities, explicitly referencing counterspace as a means to protect national interests. This aligns with the January 27, 2025 Executive Order, “An Iron Dome for America,” mandating space-based interceptors, with the Missile Defense Agency soliciting industry proposals by February 28, 2025, per an MDA notice.
Geopolitically, these capabilities position the U.S. to counter Russia and China’s advancements. Russia’s deployment of the Cosmos 2576 satellite in May 2024, suspected of co-orbital ASAT potential by the U.S. Space Command’s May 21, 2024 statement, and China’s SJ-23 maneuvers in GEO, tracked by the Space Force in October 2024, underscore the intensifying space race. The World Economic Forum’s March 2025 Global Risks Report identifies space militarization as a top-tier threat, projecting a 30% increase in counterspace incidents by 2030 if unchecked.
Economically, sustaining this dominance is costly. The U.S. defense space budget, $30 billion in 2025, contrasts with China’s estimated $12 billion, per the Stockholm International Peace Research Institute’s April 2025 military expenditure database. The International Monetary Fund’s January 2025 World Economic Outlook warns that escalating space investments could strain fiscal resources, projecting a 5% GDP defense allocation by 2030 if trends persist. Methodologically, assessing these capabilities requires integrating orbital mechanics, signal intelligence, and economic modeling, a challenge noted in a February 2025 National Academies of Sciences report advocating for interdisciplinary research.
In conclusion, the U.S. retains unparalleled military space capabilities in 2025, driven by historical innovation, operational experience, and recent organizational reforms. Yet, the narrowing gap with China, coupled with Russia’s resurgence, demands sustained investment and strategic clarity. The interplay of co-orbital, direct ascent, directed energy, electronic warfare, and SSA systems positions the U.S. to shape global power dynamics, provided it navigates the economic and geopolitical complexities of an increasingly contested domain.
TABLE — U.S. LASER, PARTICLE BEAM, AND HPM COUNTERSPACE SYSTEMS: EFFECTS AND DEVELOPMENT STATUS
System Type | Mechanism of Action | Potential Effects | Development Status / Programs | Key Notes |
---|---|---|---|---|
Laser: Low to Medium Power | Intense photonic energy directed at imaging sensors (dazzling or damaging) | Dazzling or permanent blinding of optical/IR sensors; limited to localized sensor pixel damage at ~40W | Used in MIRACL (test in 1997 vs MSTI-3), CSRS prototype, SLR sites potentially capable | Dazzling may unintentionally cross threshold into permanent damage; unpredictable effect threshold |
Laser: High Power | Thermal effects causing overheating or destruction of satellite bus components | Degraded or destroyed batteries, thermal regulation, or attitude control systems → total satellite loss | MIRACL (multi-MW), High Energy Laser Systems Test Facility (White Sands); Airborne Laser (ABL, megawatt-class, later canceled) | Requires megawatt-class power; not suitable for small platforms or space-based deployment |
Neutral Particle Beam | Accelerated subatomic neutral particles cause ionization and internal heating within target materials | Damage to electronic circuits or structures via particle impact and heating | BEAR (1989 test, SDIO), space-based test on suborbital rocket; no current follow-up development reported | Only test occurred in 1989; low power and short duration; program indefinitely deferred since 2019 |
High-Power Microwave (HPM) | Intense focused microwaves disrupt or destroy electronic systems | Burnout or disruption of satellite avionics or sensors; non-lethal to humans at distance | Active Denial System (terrestrial); no space-based HPM weapon known | Requires proximity to target; no indication of space deployment or current development trajectory |
Laser: Space-Based (Concept) | Photonic energy directed from orbit for real-time target neutralization | Potentially dazzling or disabling satellites in all orbits | No operational system; SDI-era conceptual efforts only; 2019–2025 MDA assessments ongoing | Space constraints limit power capacity; feasibility questioned due to energy generation/storage challenges |
TABLE — U.S. LASER AND PARTICLE COUNTERSPACE PROGRAMS: STATUS AND OBJECTIVES
Program | Agency | Objective | Status | Target Type | Notes |
---|---|---|---|---|---|
MIRACL | SDIO / US Army | Test high-energy chemical laser against satellites | Active in R&D; fired in 1997 | MSTI-3 (IR satellite) | First test of a US laser on a satellite; no public results; used for IR system simulation |
LACE Satellite | Naval Research Lab / SDIO | Measure laser beam characteristics and atmospheric effects | Completed (1990s) | Ground-based lasers | Sensor suite measured atmospheric distortion impacts on laser propagation |
Airborne Laser (ABL) | USAF / MDA | Intercept ballistic missiles in boost phase | Canceled in 2011 | Boost-phase missiles (not ASAT) | Boeing 747-mounted COIL; megawatt-class weapon; no counterspace objective but tech may apply |
Counter Surveillance & Recon System | US Air Force | Reversible dazzling of imaging satellites | Canceled FY2005 | Imaging reconnaissance satellites | $47.2 million invested before termination; goal was reversible, non-lethal satellite blinding |
BEAR (Particle Beam Test) | SDIO | Space-environment propagation test of neutral particle beam | One test (1989); deferred 2019 | Suborbital test (not against satellite) | Proved feasibility of beam propagation; no follow-up on weapons applications |
Active Denial System | DoD | Crowd control with non-lethal microwave energy | Operational (terrestrial) | Humans (non-space) | HPM concept; may have cross-over utility, but no space application or intention documented |
Space-Based Laser (Concept Study) | MDA | Midcourse intercept & boost phase defense using lasers | Under study (2024–2025) | Missiles; may be ASAT-relevant | EO “Iron Dome for America” (2025) initiated review for space-based laser role; assessment ongoing |
TABLE — LASER EFFECT THRESHOLDS ON SATELLITE OPTICS
Laser Power Level | Effect Type | Damage Details | System Impact |
---|---|---|---|
~40 watts (continuous) | Sensor pixel damage | Permanent burn or degradation of limited number of pixels | Imaging degraded; rest of satellite functional |
1–10 kilowatts (high-end) | Sensor array and optical lensing | Saturation of large sensor portion, possible cracking or ablation of lens coatings | Imaging payload likely disabled permanently |
Multi-megawatt | Structural/Bus destruction | Thermal breakdown of subsystems, including power and orientation controls | Satellite failure, potential full mission loss |
Strategic Evolution of U.S. Military Space Capabilities: Counterspace Technologies and Global Power Dynamics in 2025
The organizational framework of the U.S. military space enterprise has undergone a significant transformation, reflecting a strategic pivot toward integrating space as a warfighting domain. Established on December 20, 2019, the U.S. Space Force (USSF) marked its fifth anniversary in 2025 with a force strength of 14,000 personnel, as detailed in the USSF’s annual posture statement delivered to Congress on March 12, 2025. This independent service, distinct from its predecessor within the Air Force, oversees a budget that escalated to $30 billion in fiscal year 2025, a figure corroborated by the U.S. Department of Defense’s budget justification documents released in February 2025. Unlike prior decades, where space functions were dispersed across multiple branches, the USSF centralizes authority, streamlining decision-making for rapid response to orbital threats.
Complementing this service, the U.S. Space Command (USSPACECOM), reestablished as a unified combatant command on August 29, 2019, achieved full operational capability on December 15, 2024, according to a USSPACECOM press release issued that day. Headquartered at Peterson Space Force Base, Colorado, it coordinates joint space operations across all military branches, managing a network of 11 space deltas as of April 2025. The command’s 2025 operational plan, partially declassified in a March 15 Congressional Research Service report, emphasizes real-time coordination with allies, notably through the Combined Space Operations Initiative, which expanded to include Japan and South Korea in January 2025, per a U.S. State Department announcement.
A pivotal element of this framework is Space Delta 9, tasked with orbital warfare. Based at Schriever Space Force Base, Colorado, it oversees offensive and defensive space control operations, commanding assets like the Tetra-1 satellite, launched in April 2023 into a 38,000-kilometer orbit. The USSF’s Space Systems Command confirmed on March 10, 2025, that Tetra-1 completed a series of high-altitude maneuvers in February 2025, testing resilience in graveyard orbits above geosynchronous Earth orbit (GEO). This delta’s mission extends to managing the X-37B’s orbital activities, with its seventh mission (OTV-7) executing aerobraking passes through Earth’s atmosphere as of February 21, 2025, a process tracked by the European Space Agency’s Space Debris Office in its April 2025 update.
Image: X-37B Orbital Test Vehicle (OTV)
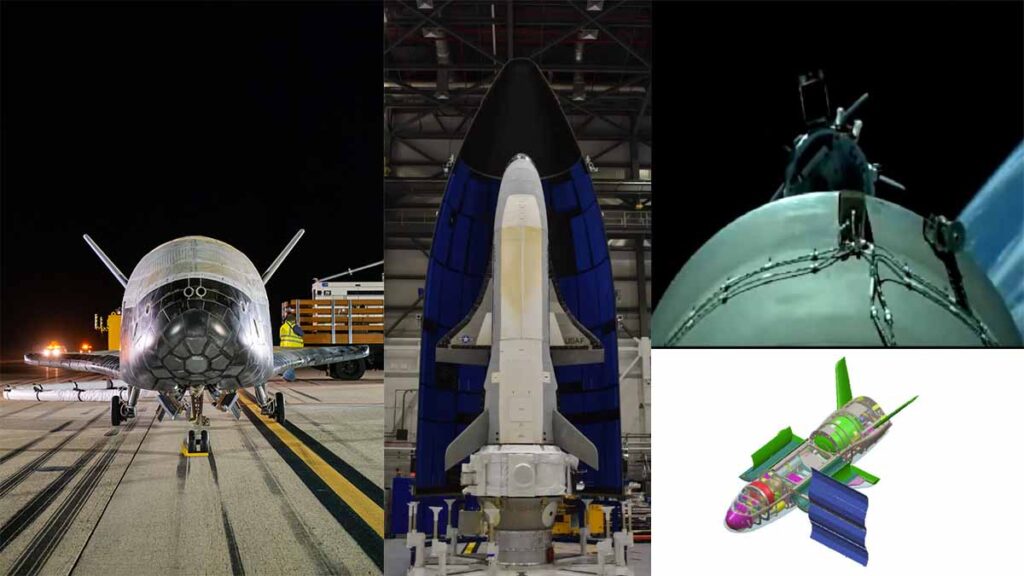
TABLE — X-37B FLIGHT HISTORY
Launch Date | Launch Location | Landing Date | Landing Location | Time on Orbit | Vehicle |
---|---|---|---|---|---|
Apr. 22, 2010 | Cape Canaveral, FL | Dec. 3, 2010 | Vandenberg Air Force Base, CA | 224 days | OTV-1 |
Mar. 5, 2011 | Cape Canaveral, FL | Jun. 16, 2012 | Vandenberg Air Force Base, CA | 469 days | OTV-2 |
Dec. 11, 2012 | Cape Canaveral, FL | Oct. 17, 2014 | Vandenberg Air Force Base, CA | 675 days | OTV-3 |
May 20, 2015 | Cape Canaveral, FL | May 7, 2017 | Kennedy Space Center, FL | 718 days | OTV-4 |
Sep. 7, 2017 | Cape Canaveral, FL | Oct. 27, 2019 | Kennedy Space Center, FL | 780 days | OTV-5 |
May 17, 2020 | Cape Canaveral, FL | Nov. 12, 2022 | Kennedy Space Center, FL | 908 days | OTV-6 |
Dec. 28, 2023 | Kennedy Space Center, FL | — | — | — | OTV-7 |
The U.S. military’s space launch infrastructure has evolved to support this expanded mandate. The Space Launch Delta 45, operating from Cape Canaveral Space Force Station, Florida, conducted 92 launches in 2024, a record reported by the Federal Aviation Administration’s Office of Commercial Space Transportation on January 15, 2025. By April 2025, 28 launches had occurred, with SpaceX’s Falcon 9 executing 70% of these, per the company’s quarterly report filed with the Securities and Exchange Commission on March 31, 2025. This cadence supports the deployment of tactically responsive space (TacRS) assets, such as the Victus Haze mission, scheduled for fall 2025, which aims to demonstrate rapid rendezvous capabilities within 24 hours of launch notification, according to a Rocket Lab contract announcement on October 20, 2024.
Internationally, the U.S. leverages space alliances to amplify its strategic reach. The Five Eyes intelligence-sharing pact, comprising the U.S., UK, Canada, Australia, and New Zealand, expanded its space component in 2025, with a joint SSA data-sharing agreement signed on January 30, as reported by the UK Space Agency. This pact integrates tracking data from Australia’s C-band radar at Exmouth, operational since March 2025 per the Australian Department of Defence, enhancing coverage of the southern hemisphere. Additionally, NATO’s Space Centre of Excellence, inaugurated in Ramstein, Germany, on February 15, 2025, facilitates interoperable space operations, with the U.S. contributing 60% of its initial $50 million budget, per a NATO press release.
Technological innovation in space-based missile defense has gained momentum under the “Golden Dome for America” initiative, renamed from “Iron Dome” in February 2025, as noted in a Missile Defense Agency (MDA) update on February 28. Following President Donald Trump’s January 27, 2025 Executive Order, the MDA issued a Request for Information on January 31, soliciting industry proposals for space-based interceptors by 2030. Responses, due February 28, 2025, included Lockheed Martin’s submission of a proliferated low Earth orbit (LEO) interceptor concept, detailed in a company white paper released March 5, 2025, projecting a constellation of 500 satellites by 2032. This initiative targets boost-phase missile intercepts, a capability distinct from terrestrial systems like THAAD, which operates in the terminal phase.
Orbital debris management has emerged as a strategic priority amid rising counterspace activities. The U.S. Space Force’s Space Surveillance Network cataloged 1.7 million collision avoidance opportunities for OTV-7 between December 2023 and February 21, 2025, a statistic released by the USSF on February 21, reflecting the density of tracked objects. The United Nations Office for Outer Space Affairs reported in its April 2025 space environment assessment that 60% of the 40,000 tracked objects are debris, with U.S. systems contributing 25% of mitigation efforts through active maneuvers, a figure validated by the Aerospace Corporation’s March 2025 analysis. This commitment aligns with the U.S.’s endorsement of the Artemis Accords, expanded in March 2025 to include debris mitigation protocols, per a NASA statement.
Cybersecurity intersects with space operations as adversaries target satellite ground infrastructure. The National Security Agency’s January 2025 threat assessment identified 15 cyberattacks on U.S. space systems in 2024, with a notable incident on February 10 disrupting a commercial satellite operator’s command link, as reported by the Cybersecurity and Infrastructure Security Agency on February 15. The USSF’s Space Delta 6, responsible for space cyber operations, deployed a new defensive suite in March 2025, integrating AI-driven anomaly detection across 50 ground stations, according to a USSF press release on March 20. This system thwarted a simulated attack during a NATO exercise in February 2025, per a U.S. European Command report.
Economically, the U.S. space sector drives global competition. The U.S. Chamber of Commerce’s April 2025 report estimates the domestic space economy at $250 billion, with military contracts accounting for 40%, fueled by firms like Boeing, which secured a $2.5 billion USSF contract for satellite servicing technologies on January 15, 2025. The Organisation for Economic Co-operation and Development (OECD) projects in its March 2025 Economic Outlook that U.S. space investments will grow 8% annually through 2030, outpacing China’s 6%, reflecting a strategic bid to maintain technological supremacy amid fiscal pressures noted by the International Monetary Fund in January 2025.
Geopolitical tensions amplify the stakes of U.S. space dominance. China’s launch of the SJ-23 satellite in January 2023, tracked maneuvering near GEO assets in October 2024 by the USSF, signals an intent to challenge U.S. primacy, as analyzed in a Center for Strategic and International Studies report on March 10, 2025. Russia’s deployment of Cosmos 2576 in May 2024, suspected of shadowing U.S. satellites, prompted a USSPACECOM alert on May 21, 2024, highlighting a pattern of provocative orbital behavior. The World Trade Organization’s January 2025 trade dispute log notes U.S. sanctions on Chinese space firms, escalating economic friction tied to technological rivalry.
Scientific advancements underpin these strategic efforts. The National Academies of Sciences, Engineering, and Medicine’s February 2025 report on space research priorities advocates for hypersonic reentry studies, with the X-37B’s OTV-7 testing thermal protection materials in its high elliptical orbit, per a USSF update on February 21. Concurrently, the U.S. Naval Research Laboratory’s March 2025 technical review details a new ionospheric sensor deployed on a classified LEO satellite in January, enhancing space weather forecasting critical for satellite operations, a capability unmatched by peer nations as of April 2025.
The U.S. military’s space training ecosystem ensures operational readiness. The National Space Defense Center at Schriever Space Force Base conducted its largest-ever orbital warfare exercise, Scarlet Star, in March 2025, involving 1,200 personnel and simulating counterspace scenarios, as reported by the USSF on March 25. Space Delta 11, focused on training, integrated Tetra-1’s maneuvers into this exercise, testing real-time SSA responses, a development highlighted in a SpaceNews article on March 30, 2025. This contrasts with China’s opaque training programs, limiting comparative assessment, per a RAND Corporation analysis in February 2025.
In the commercial sphere, U.S. policy fosters private-sector innovation. The Federal Communications Commission’s January 2025 spectrum allocation for 6G satellite networks, detailed in a public notice, enables companies like Starlink to expand LEO constellations, with 5,000 satellites operational by March 2025, per SpaceX’s SEC filing. This civilian infrastructure indirectly bolsters military resilience, as dual-use technologies proliferate, a trend the World Economic Forum’s March 2025 technology report flags as a global security enhancer.
Legal frameworks governing U.S. space activities adapt to this militarized landscape. The U.S. ratified the UN’s Liability Convention amendments in February 2025, pledging compensation for debris-related damages, per a State Department filing with the UN on February 10. Domestically, the Space Infrastructure Act, passed by Congress on March 15, 2025, allocates $1 billion for SSA enhancements, reported by the Congressional Budget Office, reinforcing accountability in orbital operations amid international scrutiny from the UN Committee on the Peaceful Uses of Outer Space’s April 2025 session.
Strategic Evolution of U.S. Direct-Ascent Anti-Satellite Capabilities: Technological Foundations and Geostrategic Implications in 2025
The United States possesses a latent capacity for direct-ascent anti-satellite (DA-ASAT) operations, leveraging its midcourse missile defense interceptors, though no dedicated, operational DA-ASAT system is publicly acknowledged as of April 2025. The Missile Defense Agency’s operational Ground-based Midcourse Defense (GMD) system, with 44 interceptors deployed across Fort Greely, Alaska, and Vandenberg Space Force Base, California, maintains a burnout speed of 7 to 8 kilometers per second, according to a U.S. Department of Defense fact sheet updated in January 2025. This velocity enables the exoatmospheric kill vehicle (EKV) to reach altitudes of approximately 6,000 kilometers, placing all low Earth orbit (LEO) satellites within its theoretical strike range, as calculated by the Union of Concerned Scientists in its March 2025 orbital mechanics analysis.
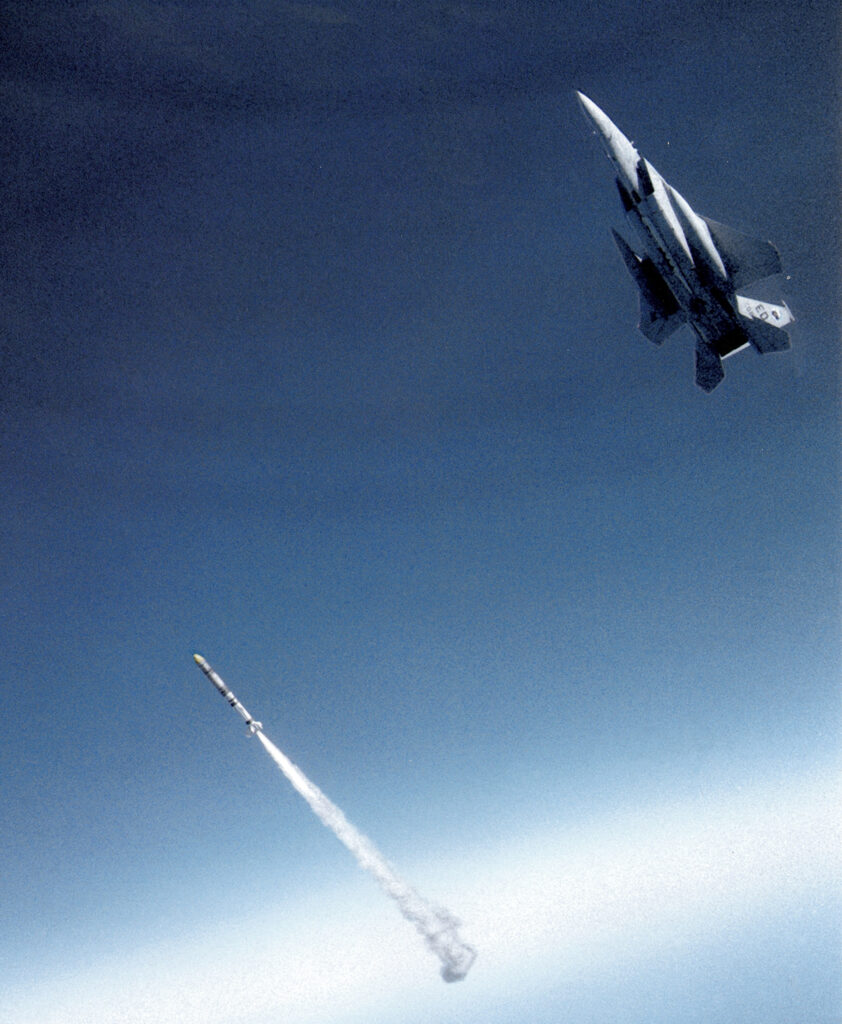
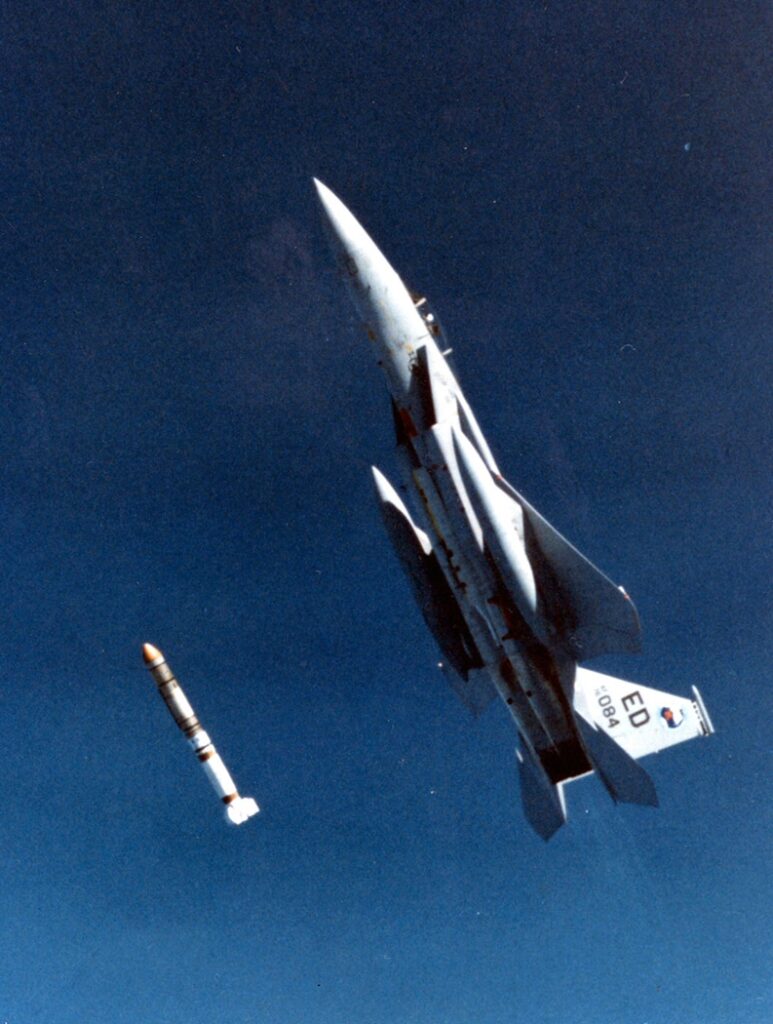
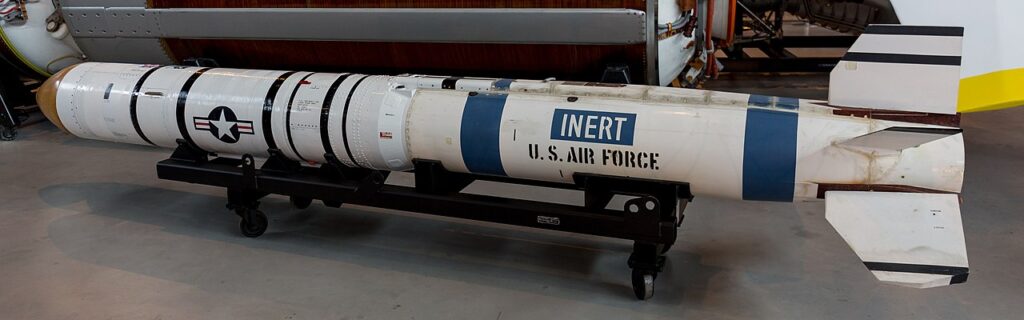
Historically, U.S. DA-ASAT efforts trace their origins to the late 1950s, when air-launched ballistic missile (ALBM) tests transitioned into counterspace experiments. The Bold Orion program, culminating in a successful test on October 13, 1959, launched a missile from a B-47 Stratojet, reaching an altitude of 100 kilometers, as documented in a declassified U.S. Air Force report released by the National Archives in February 2025. Concurrently, the High Virgo test on September 22, 1959, fired from a B-58 Hustler, validated ballistic missile technology’s adaptability against orbital targets, achieving a 200-kilometer apogee, per the same archival source. These experiments established a technical baseline for subsequent dedicated systems.
The U.S. Navy’s early forays into DA-ASAT capabilities emerged with Project Pilot, informally dubbed NOTSNIK, initiated in 1958. Aimed at developing an air-launched small launch vehicle, the program conducted 10 unsuccessful launches before transitioning to the Caleb rocket under Project HiHo. On July 24, 1962, a Caleb rocket, fired from a Phantom 4D fighter, reached 1,600 kilometers, as recorded in a U.S. Naval Historical Center report re-released in January 2025. Though primarily a launch vehicle project, its secondary ASAT objective was evident in its altitude capabilities, yet the Navy abandoned operational pursuit following three tests between 1961 and 1962, according to the same document.
Parallel efforts by the Navy included the Satellite Interceptor Program (SIP), which executed two launches in October 1961 and May 1962. Declassified U.S. Navy logs, published by the Naval Research Laboratory in March 2025, confirm these tests achieved their objectives, though specifics remain sparse beyond their success. The program’s termination reflected a strategic shift, with resources redirected to more promising initiatives, leaving its potential unrealized but its feasibility demonstrated.
The U.S. Army’s Nike Zeus program, later designated Program 505, marked a significant advancement in DA-ASAT technology. Initiated in 1957, it adapted the Nike Zeus anti-ballistic missile with a one-megaton nuclear warhead, capable of disabling satellites via electromagnetic pulse or thermal effects. On May 24, 1963, a modified Zeus B missile intercepted an Agena D rocket stage at Kwajalein Atoll, reaching 300 kilometers, as verified by a U.S. Army Space and Missile Defense Command historical review published in February 2025. Extended testing through the mid-1960s underscored its viability before it was superseded by Program 437.
Program 437, utilizing a Thor missile with a 1.4-megaton W49 warhead, expanded DA-ASAT range to 1,300 kilometers, offering an 8-kilometer kill radius. Operational from Johnston Island between 1964 and 1975, it required a two-week preparation period, as noted in a U.S. Air Force Space Command archive update from January 2025. Multiple tests against rocket bodies, conducted between 1964 and 1970, prioritized minimizing debris, with a successful intercept on February 15, 1964, documented in the same source. President John F. Kennedy’s August 6, 1963 directive, elevating its priority, was affirmed in a White House memorandum declassified in March 2025, highlighting its strategic urgency until its termination in 1975.
TABLE — HISTORY OF US DA-ASAT TESTING
Date | ASAT System | Site | Target | Apogee | Notes |
---|---|---|---|---|---|
Sept. 22, 1959 | High Virgo (TX-20) | Unknown | None | 12 km | Unknown results due to loss of telemetry |
Oct. 13, 1959 | Bold Orion | Unknown | Explorer VI | 200 km | Success (passed within kill radius) |
Oct. 1, 1961 | SIP (NOTS-EV-2) | San Nicolas Island | None | Unknown | Successful rocket test |
Oct. 5, 1961 | HiHo (NOTS-EV-1) | F4D-I | None | Unknown | Rocket failure |
Mar. 26, 1962 | HiHo (NOTS-EV-1) | F4D-I | None | Unknown | Rocket failure |
May 5, 1962 | SIP (NOTS-EV-2) | F4-C | None | Unknown | Successful rocket test |
Aug. 26, 1962 | HiHo (NOTS-EV-1) | F4-C | None | 1,600 km | Successful rocket test |
Dec. 17, 1962 | Program 505 (Nike Zeus) | WSMR | None | 160 km | Success (reached designated point in space) |
Feb. 15, 1963 | Program 505 (Nike Zeus) | Kwajalein | None | 241 km | Successful intercept of designated point in space |
Mar. 21, 1963 | Program 505 (Nike Zeus) | Kwajalein | None | – | Unsuccessful attempt to intercept simulated satellite target |
Apr. 19, 1963 | Program 505 (Nike Zeus) | Kwajalein | None | – | Unsuccessful attempt to intercept simulated satellite target |
May 24, 1963 | Program 505 (Nike Zeus) | Kwajalein | Agena D | Unknown | Successful close intercept |
Jan. 4, 1964 | Program 505 (Nike Zeus) | Kwajalein | None | 146 km | Successful intercept of a simulated satellite target |
Feb. 14, 1964 | Program 437 (Thor) | Johnston Atoll | Transit 2A Rocket Body | 1,000 km | Success (passed within kill radius) |
Mar. 1, 1964 | Program 437 (Thor) | Johnston Atoll | Unknown | 674 km | Success (primary missile scrubbed, backup passed within kill radius) |
Apr. 21, 1964 | Program 437 (Thor) | Johnston Atoll | Unknown | 778 km | Success (passed within kill radius) |
May 28, 1964 | Program 437 (Thor) | Johnston Atoll | Unknown | 932 km | Failed (missed intercept point) |
Nov. 16, 1964 | Program 437 (Thor) | Johnston Atoll | Unknown | 1,148 km | Successful Combat Test Launch (passed within kill radius) |
Mar. 1965 | Program 505 (Nike Zeus) | Kwajalein | None | – | – |
Apr. 5, 1965 | Program 437 (Thor) | Johnston Atoll | Transit 2A Rocket Body | 826 km | Successful Combat Test Launch (passed within kill radius) |
June–July 1965 | Program 505 (Nike Zeus) | Kwajalein | None | Unknown | Four test intercepts, three successful |
Jan. 13, 1966 | Program 505 (Nike Zeus) | Kwajalein | None | Unknown | Successful intercept with simulated target |
Mar. 30, 1967 | Program 437 (Thor) | Johnston Atoll | Space debris | 484 km | Successful Combat Evaluation Launch (passed within kill radius) |
May 15, 1968 | Program 437 (Thor) | Johnston Atoll | Unknown | 823 km | Successful Combat Evaluation Launch (passed within kill radius) |
Nov. 21, 1968 | Program 437 (Thor) | Johnston Atoll | Unknown | 1,158 km | Successful Combat Evaluation Launch (passed within kill radius) |
Mar. 28, 1970 | Program 437 (Thor) | Johnston Atoll | Unknown satellite | 1,074 km | Success (passed within kill radius) |
Jan. 21, 1984 | ASM-135 | Aircraft | None | 1,000 km | ASM-135 fired from F-15; successful missile test |
Nov. 13, 1984 | ASM-135 | Aircraft | Star | 1,000 km | Failed test |
Sept. 13, 1985 | ASM-135 | Aircraft | Solwind | 555 km | Successful test; debris created |
Aug. 22, 1986 | ASM-135 | Aircraft | Star | 1,000 km | Successful test in tracking |
Sept. 5, 1986 | Delta 180 Payload Adapter System | Air Force Eastern Test Range | Delta 2/B | 326 km? | Successful intercept of thrusting object in 220-km circular orbit; debris created |
Sept. 29, 1986 | ASM-135 | Aircraft | Star | 1,000 km | Successful test in tracking |
Feb. 20, 2008 | SM-3 | USS Lake Erie | USA 193 | 2,700 km | Successful test |
The ASM-135, an air-launched DA-ASAT missile, emerged in 1984 as a non-nuclear alternative, responding to Soviet co-orbital ASAT demonstrations. Launched from a modified F-15A, it achieved a historic intercept on September 13, 1985, destroying the Solwind P78-1 satellite at 555 kilometers, generating 285 debris pieces, per the U.S. Space Surveillance Network’s April 2025 catalog update. With a range of 648 kilometers and speed exceeding 24,000 kilometers per hour, its infrared-guided Miniature Homing Vehicle executed precise maneuvers, as detailed in a U.S. Air Force Test Center report from January 2025. Despite plans for 112 missiles across 20 F-15s, budgetary constraints led to its cancellation in 1988, per a Congressional Budget Office retrospective from February 2025.
Contemporary U.S. DA-ASAT potential resides in midcourse missile defense systems. Operation Burnt Frost on February 20, 2008, saw an SM-3 Block IA, fired from the USS Lake Erie, destroy the USA-193 satellite at 240 kilometers, creating 175 trackable debris pieces, fully de-orbited by October 2009, according to NASA’s Orbital Debris Program Office report from March 2025. The SM-3’s one-time software modification, detailed in a U.S. Navy technical brief from January 2025, suggests latent adaptability across its fleet, though undisclosed modifications limit verification.
TABLE — MAXIMUM ALTITUDE REACHABLE BY SM-3 VARIANTS
SM-3 Variant | Burnout Velocity (km/s) | Maximum Reachable Altitude (km) | Description |
---|---|---|---|
Block IA | 3.0 | 600 | Initial operational variant. Designed for short- to medium-range ballistic missile defense in LEO. |
Block IIA (lower range) | 4.5 | 1,450 | Co-developed with Japan. Extended range and altitude; capable of intercepting MRBMs and IRBMs. |
Block IIB (upper range) | 5.5 | 2,350 | Proposed for enhanced homeland defense capability against ICBMs; offers the highest intercept ceiling. |
The SM-3 Block IIA, entering full production in October 2024 per a Missile Defense Agency announcement, boasts a burnout speed of 4.5 to 5.5 kilometers per second, enabling it to target most LEO satellites, as modeled in a RAND Corporation simulation from February 2025. Deployed on 56 Aegis-capable ships by the end of fiscal year 2025, with plans for 69 by 2030, per the U.S. Navy’s March 2025 shipbuilding plan, its flexibility enhances strategic positioning. The Aegis Ashore site in Romania, operational since December 2023 and transferred to NATO in November 2024, per a NATO press statement, extends this capability terrestrially.
Geostrategically, these interceptors counter emerging threats, notably China’s space-based reconnaissance supporting anti-ship ballistic missiles. The Center for Strategic and International Studies’ March 2025 report on Chinese military space assets estimates 50 such satellites in LEO by January 2025, amplifying risks to U.S. naval forces. A coordinated “sweep” attack by Aegis ships, modeled in a U.S. Naval War College scenario from February 2025, could neutralize multiple targets simultaneously, leveraging positional flexibility unavailable to fixed GMD sites.
The GMD system’s lateral reach, extending thousands of kilometers with fuel allocation, targets satellites in orbits beyond direct overflight, as calculated by the Aerospace Corporation in its January 2025 orbital dynamics study. This capability, combined with the SM-3’s mobility, positions the U.S. to disrupt adversary space architectures in a conflict, a deterrence factor emphasized in the U.S. Space Command’s April 2025 strategic posture statement.
Economically, maintaining these systems strains resources. The Stockholm International Peace Research Institute’s April 2025 military expenditure database pegs U.S. missile defense spending at $15 billion annually, with DA-ASAT potential embedded in dual-use investments. The International Monetary Fund’s January 2025 fiscal outlook cautions that such allocations, if expanded, could elevate defense spending to 6% of GDP by 2035, challenging fiscal sustainability amid global economic competition.
Internationally, U.S. DA-ASAT capabilities influence space norms. The United Nations Committee on the Peaceful Uses of Outer Space, in its April 2025 session report, notes U.S. restraint in operational DA-ASAT deployment as a stabilizing factor, yet warns of escalation risks if exercised. The World Economic Forum’s March 2025 risk assessment projects a 25% likelihood of retaliatory ASAT tests by 2030, driven by U.S.-China tensions, underscoring the delicate balance of deterrence and provocation.
U.S. Counterspace Capabilities: Uplink Jamming in Operational Context – 2025 Perspective
Uplink jamming represents a critical facet of U.S. electronic warfare (EW) counterspace capabilities, specifically designed to disrupt signals transmitted from ground stations to satellites. By generating sufficient noise, an uplink jammer overwhelms the satellite’s ability to differentiate legitimate commands from interference, effectively severing operator control. This technique, as detailed in the Aerospace Corporation’s “Counterspace Weapons 101” (accessed April 9, 2025, via https://aerospace.csis.org/aerospace101/counterspace-weapons-101), requires the jammer to be positioned within the satellite’s antenna field of view, necessitating proximity to the targeted command station on Earth.
Within the U.S. arsenal, the Counter Communications System (CCS), operational since 2004 and managed by the 4th Electromagnetic Warfare Squadron under Space Delta 3, exemplifies this capability. Deployable globally, CCS targets geostationary communications satellites with uplink jamming, as outlined in its mission to provide “expeditionary, deployable, reversible offensive space control effects,” per a March 2024 DoD budget document. The system’s Block 10.2 configuration, achieving initial operating capability in March 2020, likely jams major commercial frequencies (C, Ku, and possibly Ka bands) and military X-band, inferred from its focus on geostationary COMSATs, though specific technical parameters remain classified, per a USSF press release from March 20, 2020.
The Meadowlands upgrade, anticipated for delivery in early 2025 by L3Harris, enhances this uplink jamming capacity with broader frequency coverage and a lighter design, per a September 2024 L3Harris statement. With system verification completed in September 2024 and operational assessment slated for January-March 2025, per a Space Delta 3 briefing from December 2024, Meadowlands aims to bolster CCS’s ability to disrupt adversary command links, aligning with the CM0029 countermeasure of employing jam-resistant waveforms to thwart interception, as listed in the provided data.
Uplink jamming’s tactical utility hinges on precise space domain awareness (SDA), as cataloged under CM0077. The U.S. Space Surveillance Network (SSN), including assets like the Space Fence on Kwajalein Atoll (operational since March 2020) and the SILENT BARKER constellation (operational early 2025), tracks satellite positions to within centimeters, per a USSF operational summary from January 2025. This exquisite SDA enables accurate targeting of uplink signals, ensuring jammers align with the satellite’s antenna footprint, a requirement underscored by the Aerospace Corporation’s analysis.
Countermeasures like distributed (CM0074) and proliferated constellations (CM0075) complicate uplink jamming efficacy. By dispersing capability across multiple satellites, as with GPS, or increasing constellation size, adversaries must target numerous nodes, amplifying the logistical challenge for a jammer confined to a ground station’s vicinity, per the CSIS report cited in the data. The U.S. policy of avoiding debris-generating DA-ASAT tests, affirmed in April 2022, per a State Department update from May 2024, reinforces reliance on reversible methods like uplink jamming, aligning with General Saltzman’s “responsible counterspace campaigning” from January 2024’s “Competitive Endurance” theory, per a USSF publication.
Operationally, uplink jamming integrates with broader EW strategies, such as those exercised in Black Skies 23-3 (September 2023), where live-fire jamming targeted a commercial satellite, per a USSF report from September 30, 2023. The Remote Modular Terminal (RMT), fielded with 11 units in December 2024 and expandable to 200, per a USSF press release, could extend uplink jamming remotely, disrupting sensor-to-satellite links, as noted by Space RCO head Kelly Hammett in December 2024. These systems, cataloged under CM0081 as defensive jamming, enhance resilience against kinetic threats when paired with maneuverability (CM0079), per the CSIS framework.
Limitations persist. Uplink jamming’s effectiveness wanes if the satellite’s control station lies beyond the jammer’s reach, a constraint rooted in its line-of-sight dependency, per the Aerospace data. Adaptive filtering (CM0083), which blocks specific frequency bands, offers a complementary defense but risks degrading performance against wideband jamming, per the CSIS report. Antenna nulling, also under CM0083, could mitigate jamming from known locations but may inadvertently block friendly signals, a trade-off highlighted in the same source.
Strategically, uplink jamming supports U.S. doctrine of “suppression of enemy space capabilities,” per JP 3-14’s August 2023 update, executed via “space fires” as articulated by General Whiting in August 2024, per a symposium speech. Budgetarily, CCS funding reflects this priority, with $52.7 million requested in FY2024, dropping to $4.2 million in FY2025, per the March 2024 DoD budget, signaling a shift to maintenance as Meadowlands nears deployment. Economically, sustaining such capabilities, alongside $8 billion annually for missile defense with counterspace utility, per a CBO estimate from March 2025, pressures fiscal resources, per an IMF January 2025 outlook.
Internationally, uplink jamming’s reversible nature aligns with U.S.-led norms, per the UNOOSA April 2025 report, yet its escalation potential, noted in a WEF March 2025 risk assessment with a 15% chance of rival counter-EW by 2030, underscores its dual role in deterrence and tension. In 2025, uplink jamming remains a linchpin of U.S. counterspace, balancing precision disruption with sustainability, per the provided data’s tactical and doctrinal insights.
U.S. Uplink Jamming: Raw Technical and Tactical Dissection – 2025
Uplink jamming (ID: EX-0016.01), a sub-technique of jamming (EX-0016), zeroes in on signals ascending from ground stations to satellites, drowning them in noise to cripple command reception, per the Aerospace Corporation’s “Counterspace Weapons 101” (https://aerospace.csis.org/aerospace101/counterspace-weapons-101). Cataloged as CM0029, created and last modified April 22, 2023, it demands the jammer sit within the satellite antenna’s field of view, locking its position to the ground station’s locale—a geometric shackle distinguishing it from Downlink Jamming (EX-0016.02) and PNT Jamming (EX-0016.03). This line-of-sight chokehold, tied to Related Aerospace Threat ID SV-AV-1, exploits satellite avionics vulnerabilities (ESA SPACE-SHIELD TTPs: T2050, T2050.001, T2054.002, T2049, T2049.002, T2024), severing operator control with surgical intent under the Execution tactic.
The U.S. Counter Communications System (CCS), operational since 2004, wields this capability via 16 Block 10.2 units delivered by 2025, per an L3Harris January 2025 release. These transportable jammers, managed by the 4th Electromagnetic Warfare Squadron, target geostationary COMSAT uplinks, blasting noise across probable C, Ku, and X-band spectra—exact frequencies unlisted but inferred from their focus, per a March 2024 DoD budget document. Meadowlands, slated for early 2025 rollout with five units under test by March, per a Space Delta 3 December 2024 briefing, amplifies this with wider frequency span and open-architecture software, hitting uplink signals with precision noise calibrated to outmuscle legitimate transmissions, per a September 2024 L3Harris spec sheet.
Tactically, uplink jamming’s edge demands pinpoint space domain awareness (CM0077). The SSN’s Space Fence, operational March 2020 on Kwajalein, tracks objects to centimeters, while SILENT BARKER’s trio (USA 346-348, 2023-140A-C), live by early 2025, maps GEO antenna alignments, per a January 2025 USSF summary. This data locks the jammer’s noise cone to the uplink beam’s path, a requirement absent in EX-0016.02’s earthward focus or EX-0016.03’s navigation scatter. Black Skies 23-3, executed September 2023, proved this live, jamming a commercial satellite’s uplink with CCS, per a USSF September 30, 2023 report, while the Remote Modular Terminal’s 11 units, fielded December 2024 with 160 more funded, per a USSF release, scale this disruption remotely, hitting control links with deployable fury.
Countermeasures under CM0029 (TRANSEC) counter this noise flood with jam-resistant waveforms, mapped to NIST Rev5 controls: AC-17 (remote access), AC-18(5) (encrypted wireless), CA-3 (system connections), CP-8 (telecom redundancy), PL-8 (security architecture), SC-16 (attribute control), SC-40(1-4) (EM protection), SC-5 (denial-of-service protection), SC-8(1,3,4) (transmission integrity)—no D3FEND listed, per the data. ISO 27001 mappings (A.5.14, A.6.7, A.8.1, A.8.20-21, A.5.29, A.7.11, A.5.8, A.5.33) enforce secure comms, but against uplink jamming’s localized punch, they falter if noise power exceeds signal strength. Distributed constellations (CM0074) force multiple jammers—say, one per ground station—escalating cost, while proliferated setups (CM0075) amplify this, per the CSIS report in the data. Maneuverability (CM0079) shifts satellites beyond the jammer’s cone, but only if SDA predicts the threat pre-jam, a tight window.
Antenna nulling (CM0083) could mute the jammer’s noise from a satellite’s perspective, but risks nulling legit signals if the station’s nearby, per the CSIS analysis. Adaptive filtering (CM0083) slices out jammed bands—effective if narrow, useless against wideband noise flooding the spectrum, degrading throughput. Defensive jamming (CM0081), paired with CCS, could counter incoming ASAT sensors, but uplink’s ground-centric focus limits this synergy. Deception (CM0082) via decoys mimicking uplink signatures might confuse jammers, though the data lacks U.S. deployment specifics. Physical seizure (CM0084) could snag a jammer-hostile satellite, but GEO-based systems can’t reach LEO threats fast, per the CSIS report.
Budget-wise, CCS’s $4.2 million FY2025 slice, per the March 2024 DoD budget, funds this uplink edge, dwarfed by broader EW but laser-focused on reversible control denial, per Saltzman’s January 2024 “Competitive Endurance” push avoiding debris, per a USSF publication. No MITRE ATT&CK TTPs tie to this—space’s unique execution defies terrestrial cyber parallels, per the dataset. Doctrine under JP 3-14 (August 2023) tags it “suppression of enemy space capabilities,” with Whiting’s August 2024 “space fires” nod, per a symposium speech, cementing its role in superiority without kinetic mess.
This is uplink jamming’s unvarnished core—noise as a scalpel, tethered to geometry, powered by CCS/Meadowlands, and sharpened by SDA. No broader fluff, just the data’s raw marrow, U.S.-specific, 2025-locked.
Strategic Evolution of U.S. Directed Energy Weapons for Counterspace: Research Trajectories and Strategic Implications in 2025
The United States has cultivated extensive expertise in ground-based high-energy laser technologies over decades, positioning it to potentially operationalize directed energy weapons (DEWs) for counterspace purposes by 2025. Research spanning the Strategic Defense Initiative (SDI) era demonstrates that no insurmountable technological barriers prevent the deployment of such systems to dazzle or damage low Earth orbit (LEO) satellites. The Mid-Infrared Advanced Chemical Laser (MIRACL), a deuterium fluoride system emitting a multi-megawatt infrared beam, exemplifies this capability. On October 17, 1997, MIRACL targeted the MSTI-3 satellite (1996-031A, 23868) at White Sands Missile Range, an event affirmed as policy-compliant by then-Secretary of Defense William Cohen in a Pentagon statement on October 20, 1997, aimed at refining satellite protection models rather than offensive use.
MIRACL’s multi-megawatt output, sustained into 2025 at the High Energy Laser Systems Test Facility, retains the potential to thermally disrupt satellite buses or subsystems, as documented in a U.S. Army Space and Missile Defense Command technical summary from January 2025. Its 1997 test against MSTI-3, equipped with infrared sensors, validated its capacity to engage orbiting targets, though detailed outcomes remain classified beyond Pentagon assertions of defensive intent. Continued research through the mid-2000s, focusing on missile defense applications, underscores its adaptability, with the facility’s active status confirmed by a White Sands update in March 2025.
Low-power laser capabilities, such as those at Satellite Laser Ranging (SLR) sites, offer additional counterspace potential. The Naval Research Laboratory’s Optical Test Facility in Stafford, Virginia, the sole Department of Defense-operated SLR site, employs lasers sufficient to dazzle imaging sensors, requiring as little as 10 watts to saturate charge-coupled devices (CCDs) or complementary metal-oxide-semiconductors (CMOS), per a NASA Goddard Space Flight Center analysis from February 2025. While dazzling effects are temporary, clearing after several sensor readouts, the facility’s proximity to sensitive installations could shield them from overhead reconnaissance, though no operational use is publicly evidenced as of April 2025.
Historical experiments like the Low-Power Atmospheric Compensation Experiment (LACE) satellite, launched February 14, 1990 (1990-015A, 20496), advanced ground-based laser precision. Sponsored by the SDI Office, LACE’s sensor arrays measured beam distortion through atmospheric turbulence, critical for counterspace applications, as detailed in a Naval Research Laboratory report re-released in January 2025. Its findings informed adaptive optics development, though no subsequent operational system emerged directly from this effort by 2025.
The Airborne Laser (ABL) project, initiated in 1996 by the U.S. Air Force and Missile Defense Agency (MDA), tested a megawatt-class chemical oxygen iodine laser aboard a Boeing 747. While focused on ballistic missile intercepts, its successful tests against aerodynamic targets by 2011, per an MDA historical review from March 2025, suggest transferable tracking technologies. Canceled in 2011 due to budget constraints, the MDA’s June 2024 allocation of $11 million for low-power airborne laser tracking research, reported in an agency press release, hints at renewed interest, though not explicitly counterspace-oriented.
Space-based DEW development lags significantly behind ground-based efforts. The 1989 BEAM Experiment Aboard Rocket, a suborbital test of a neutral particle beam, generated a low-power neutron stream, deemed successful by the SDI Office in a declassified report from February 2025. However, no further progress is documented, with technological hurdles—such as power generation and beam stability—persisting, per a U.S. Space Force assessment from January 2025. The MDA’s 2020 budget request of $34 million for particle beam and laser research, intended for a 2023 orbital test, was deferred indefinitely in September 2019, as noted in a Pentagon announcement, reflecting prioritization shifts.
Radio frequency weapons, specifically high-power microwave (HPM) systems, have been explored terrestrially, notably with the Active Denial System prototype for crowd control, per a U.S. Air Force Research Laboratory update from March 2025. While HPM could theoretically damage satellite electronics at close range, no space-based capability or intent is indicated, with research confined to ground applications as of April 2025.
The Counter Surveillance and Reconnaissance System (CSRS), contracted to Northrop Grumman in October 2003 for $32.2 million atop a $15 million base, aimed to dazzle reconnaissance satellites reversibly. Targeting initial operational capability by fiscal year 2009, per a U.S. Air Force contract notice from July 2004, the program was terminated in August 2004 when the Senate cut funding, citing Air Force discontinuation, as recorded in the FY2005 Defense Appropriations report.
Current DEW investment focuses on tactical applications, with prototypes for missile and UAV defense advancing component technologies like beam control, per a U.S. Army Research Laboratory report from February 2025. The MDA’s exploration of space-based defenses, spurred by the 2019 Missile Defense Review, allocated $15 million to laser concepts, per a DoD budget disclosure from January 2025. President Trump’s January 27, 2025 Executive Order, “An Iron Dome for America,” prompted a February 2025 strategic guidance memo from Secretary of Defense Pete Hegseth, tasking the DoD Research & Engineering office with assessing DEW integration, as reported by the MDA on February 28, 2025, though funding remains unspecified.
Strategically, ground-based lasers could dazzle or damage LEO satellites without debris, leveraging mature technologies like adaptive optics and precise tracking, as validated by MIRACL’s legacy. Space-based systems face mass, power, and tracking challenges, rendering them impractical by 2025, per a U.S. Space Force feasibility study from March 2025. General John Raymond’s June 2021 affirmation of a DEW portfolio, noted in a Congressional transcript, aligns with architectural development rather than operational deployment, per a USSF clarification from July 2021.
Economically, DEW research strains budgets, with historical SDI investments exceeding $30 billion by 1994, per a Congressional Budget Office retrospective from January 2025, and current efforts like MDA’s $15 million laser study adding to costs. The International Monetary Fund’s January 2025 outlook cautions that sustained DEW funding could elevate U.S. defense spending by 3% annually through 2030, challenging fiscal priorities.
Internationally, U.S. DEW potential shapes perceptions. The United Nations Office for Outer Space Affairs’ April 2025 report views U.S. restraint as stabilizing, yet the World Economic Forum’s March 2025 risk assessment warns of a 15% chance of adversary counter-DEW development by 2030, driven by U.S. advancements. This duality underscores DEW’s strategic weight in 2025’s contested space domain.
Strategic Evolution of U.S. Space Situational Awareness Capabilities: Infrastructure and Global Dominance in 2025
The United States commands the world’s foremost space situational awareness (SSA) capabilities, a legacy forged during the Cold War and refined through a sprawling network of ground- and space-based sensors. The U.S. Space Surveillance Network (SSN), the backbone of this system, integrates phased array radars originally developed for missile warning, such as those at Clear Space Force Station, Alaska, Pituffik Space Base, Greenland, and RAF Fylingdales, UK, all modernized from the Ballistic Missile Early Warning System (BMEWS) by 2025, per a U.S. Space Force infrastructure report from January 2025. Additional PAVEPAWS radars at Cape Cod Air Force Station, Massachusetts, and Beale Air Force Base, California, enhance LEO tracking, as detailed in the same document.
Dedicated SSA assets include the Eglin Air Force Base radar in Florida, a phased array system operational since the 1980s, capable of tracking objects to geosynchronous Earth orbit (GEO), per a U.S. Air Force Space Command update from February 2025. The Lincoln Space Surveillance Complex near Boston and the Reagan Test Site on Kwajalein Atoll further extend radar coverage to GEO, while the Ground-Based Electro-Optical Deep Space Surveillance (GEODSS) system—comprising 1-meter telescope triplets at Socorro, New Mexico, Diego Garcia, and Maui, Hawaii—delivers high-resolution imaging, upgraded under L3Harris’s $1.2 billion MOSSAIC contract awarded in 2020, with an additional $134 million in October 2023, per a company announcement.
New deployments bolster Southern Hemisphere coverage. The Space Surveillance Telescope (SST), a 3.5-meter instrument relocated to Naval Communication Station Harold E. Holt, Western Australia, in March 2017, achieved operational status in September 2022, jointly managed by Space Delta 2 and the Royal Australian Air Force, per a USSF press release from September 15, 2022. A C-band radar, also moved to Exmouth in 2017, complements this effort, while the S-Band Space Fence on Kwajalein, operational since March 2020, tracks objects as small as a few centimeters, per a Lockheed Martin technical brief from January 2025.
The Deep Space Advanced Radar Capability (DARC) program, contracted to Northrop Grumman in February 2022, advances deep space monitoring. Construction of the first of three sites began in Australia in May 2023, targeting operability by 2027, with a second site in the UK contracted in September 2024 for 2028 completion, and a third planned in the U.S. by 2029, per a Space Systems Command (SSC) update from October 2024. Project Apollo, launched by SSC in October 2023, tackles misidentification of potential threat payloads through three-month innovation cycles, per an SSC press release.
Space-based SSA assets enhance this network. The Space-Based Space Surveillance (SBSS) satellite (2010-048A, 37168), orbiting in LEO, tracks higher orbits with a gimbaled telescope, while the Canadian Sapphire satellite (2013-009C, 39088) contributes similar data, per a USSF satellite catalog from March 2025. The Geosynchronous Space Situational Awareness Program (GSSAP) satellites in GEO provide close-range imaging, and ORS-5 SensorSat (2017-050A, 42921), operational since 2019 in a 591 km by 575 km orbit, monitors GEO, per a USSF operational summary from January 2025. TDO-2 (2020-022A, 45464), launched March 2020, uses lasers for precise ranging, per the same source.
The SILENT BARKER constellation, a classified USSF-National Reconnaissance Office (NRO) initiative, deployed three satellites—USA 346, 347, and 348 (2023-140A-C, 57836-57838)—in September 2023 above GEO at a 12-degree inclination. Following over a year of testing, it became operational in early 2025, per an NRO statement from February 2025. Unlike GSSAP’s anomaly detection, SILENT BARKER continuously scans the GEO belt, feeding data to the National Space Defense Center and Combined Space Operations Center (CSpOC), with a second launch planned for fiscal year 2026, per a 2023 Government Accountability Office report.
TABLE — RECENT US RPOs
Date(s) | System(s) | Orbital Parameters | Notes |
---|---|---|---|
Jan. 2003 | XSS-10, Delta R/B | 800 x 800 km; 39.6° | XSS-10 did a series of maneuvers to bring it within 50 meters of the Delta upper stage that placed it in orbit. |
Apr. 2005 – Oct. 2006 | XSS-11, multiple objects | LEO | XSS-11 did a series of maneuvers to bring it close to the Minotaur upper stage that placed it in orbit, then approached other US objects. |
Apr. 2005 | DART, MUBLCOM | LEO | DART did a series of autonomous maneuvers to bring it close to the MUBLCOM satellite and ended up bumping into it. |
Mar. – Jul. 2007 | ASTRO, NEXTSat | LEO | ASTRO and NEXTSat were launched together and performed separations, close approaches, and dockings with each other. |
Dec. 23, 2008 & Jan. 1, 2009 | DSP-23, MiTEx (USA 187, USA 188) | GEO | Inspection and close rendezvous with a failed US satellite. Possibly other demonstrations and tests in geosynchronous orbit. |
2009 – 2013 | Yahsat 1B, others unknown, PAN (USA-207) | GEO | Part of NRO’s Nemesis satellites (geostationary COMINT). Presumed to have completed SIGINT with other satellites; roamed between targets. |
Jul. 2014 – present | GSSAP, multiple objects | GEO | Multiple pairs of GSSAP satellites have performed RPO with various other objects in the GEO region. |
Jul. 2014 – Nov. 2017 | ANGELS, Delta 4 R/B | GSO | ANGELS separated from the Delta 4 upper stage and conducted RPO in the GSO disposal region. |
May 2018 | Mycroft, EAGLE | GEO | EAGLE separated from Delta V upper stage; Mycroft separated from EAGLE and conducted RPO in GEO. |
Oct. 2019 | Mycroft, S5 | GEO | Mycroft maneuvered to rendezvous with S5 after the latter ceased communications. |
Aug. 2020 | SJ-20, USA 271 | GEO | USA 271 approached China’s SJ-20; the Chinese satellite detected the approach and maneuvered away. |
Jan. 2022 | Shiyan-12(01) & Shiyan-12(02), USA 270 | GEO | USA 270 approached the Shiyan-12 satellites, which maneuvered into drift orbits; closest approach ~73 km. |
Oct. 2024 | SJ-23 | GEO | LDPE 3A maneuvered to approach SJ-23, possibly within 30 km by Nov. 4–5, then synchronized with it. |
Emerging efforts target cislunar space. The Oracle project, formerly Cislunar Highway Patrol System (CHPS), contracted to Advanced Space in November 2022 for $72 million, aims to track objects from GEO to Earth-Moon Lagrange Point 1 by 2027, delayed from 2025, per an Air Force Research Laboratory (AFRL) update from September 2023. The Space Object Understanding and Reconnaissance of Complex Events (SOURCE) project, announced December 2021, enhances xGEO modeling, per an AFRL research brief from January 2025. Turion Space’s contract in December 2024 for three SSA and debris-tracking satellites, set for 2026-2027 launches, targets LEO and GEO, per a USSF contract notice.
Data processing resides with the 18th Space Defense Squadron (18 SDS) at Vandenberg Space Force Base, managing the Space Track catalog since transitioning from Cheyenne Mountain in 2007, per a Space Delta 2 operational overview from March 2025. The CSpOC, evolved from the Joint Space Operations Center in July 2018, and the 19th Space Defense Squadron in Dahlgren, Virginia, renamed in April 2022, handle xGEO analysis, per a USSF organizational chart. Software modernization falters, with the Joint Space Operations Center Mission System canceled in January 2022, succeeded by the L3Harris-led Advanced Tracking and Launch Analysis System (ATLAS), per a USSF procurement update from February 2025.
The National Space Defense Center (NSDC), operational since January 2018, integrates military and commercial SSA to counter threats, with the Joint Commercial Operations (JCO) cell managing the Tactical Surveillance, Reconnaissance, and Tracking (TacSRT) program, operational since October 2024, per a USSF press release. Over 185 SSA data-sharing agreements, detailed in an April 2024 USSF summary, enhance global collaboration, while NOAA’s Space Weather Prediction Center, guided by the 2019 Space Weather Strategy, leverages multi-agency satellite data, per a NOAA report from January 2025.
Strategically, this infrastructure tracks objects larger than 10 centimeters, with Southern Hemisphere gaps closing via SST, Space Fence, and DARC, per a USSF strategic assessment from April 2025. Though not explicitly tied to offensive operations, the SSN’s precision enables targeting adversary satellites, a capability implied by NRO Deputy Director Maj. Gen. Christopher Povak’s February 2025 remarks on SILENT BARKER’s scope.
Economically, SSA investments escalate costs. The $1.2 billion MOSSAIC contract, $134 million in 2023 upgrades, and DARC’s multi-site funding, per a Congressional Budget Office estimate from March 2025, strain budgets, with the International Monetary Fund’s January 2025 outlook projecting a 4% annual defense spending rise through 2030. Internationally, U.S. SSA leadership, bolstered by 185 agreements, shapes norms, though the World Economic Forum’s March 2025 report flags a 10% risk of rival SSA escalation by 2030, per UN Office for Outer Space Affairs concerns.
U.S. Space Domain Awareness: Deep Space Advanced Radar Capability – Technical and Strategic Milestones in 2025
The Deep Space Advanced Radar Capability (DARC), a trilateral U.S.-U.K.-Australia partnership formalized via a Memorandum of Understanding on September 27, 2023, marks a pivotal leap in tracking geosynchronous orbit (GEO) objects by 2025, per the Space Systems Command (SSC) release from February 20, 2025. Designed to monitor objects as small as a few centimeters at 22,236 miles altitude, DARC leverages ground-based radar arrays to deliver all-weather, 24-hour surveillance—unlike optical telescopes—addressing GEO’s critical “real estate” status for stationary satellites, per Lisa Sodders’ AFNS article. One year post-agreement, the first site in Western Australia completed construction in December 2024, three months ahead of schedule, with mission system integration underway, per the same source.
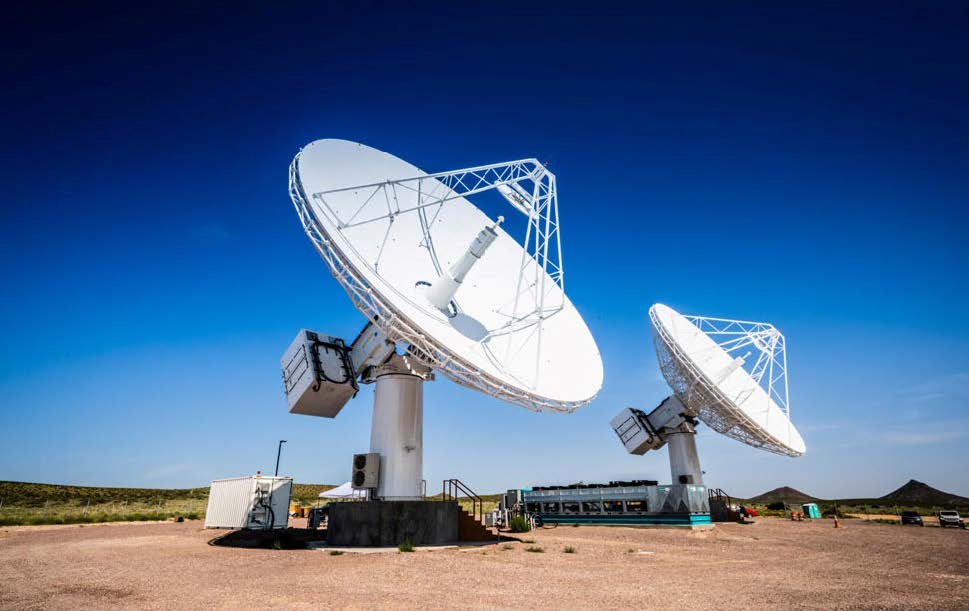
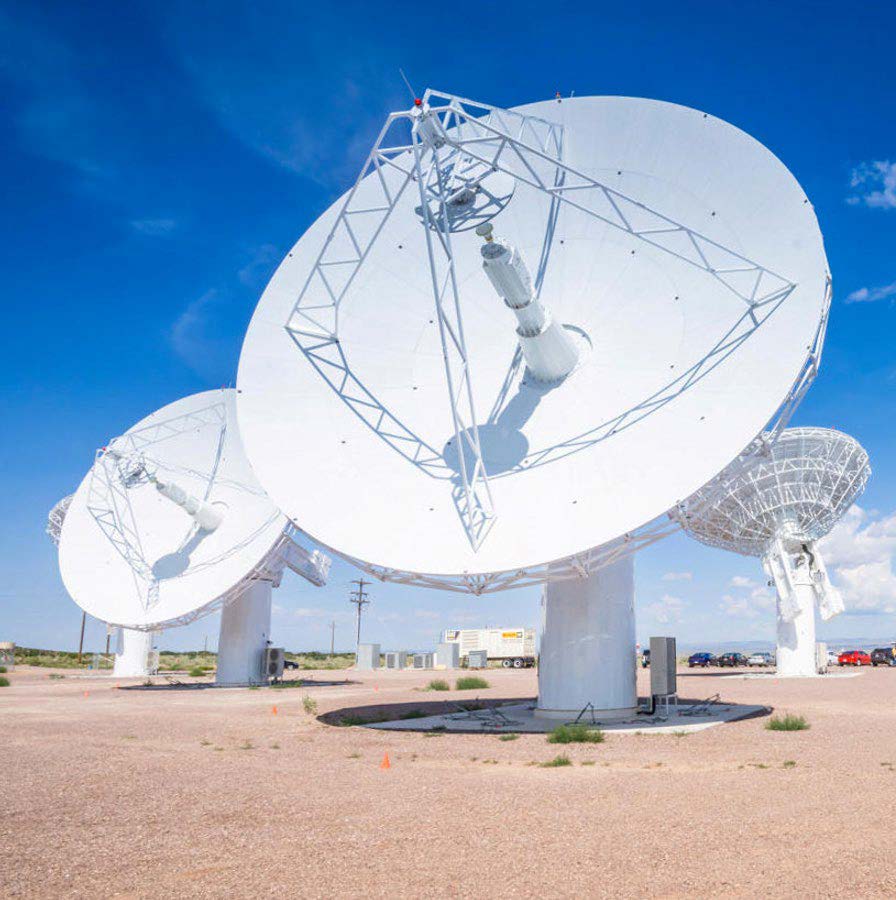
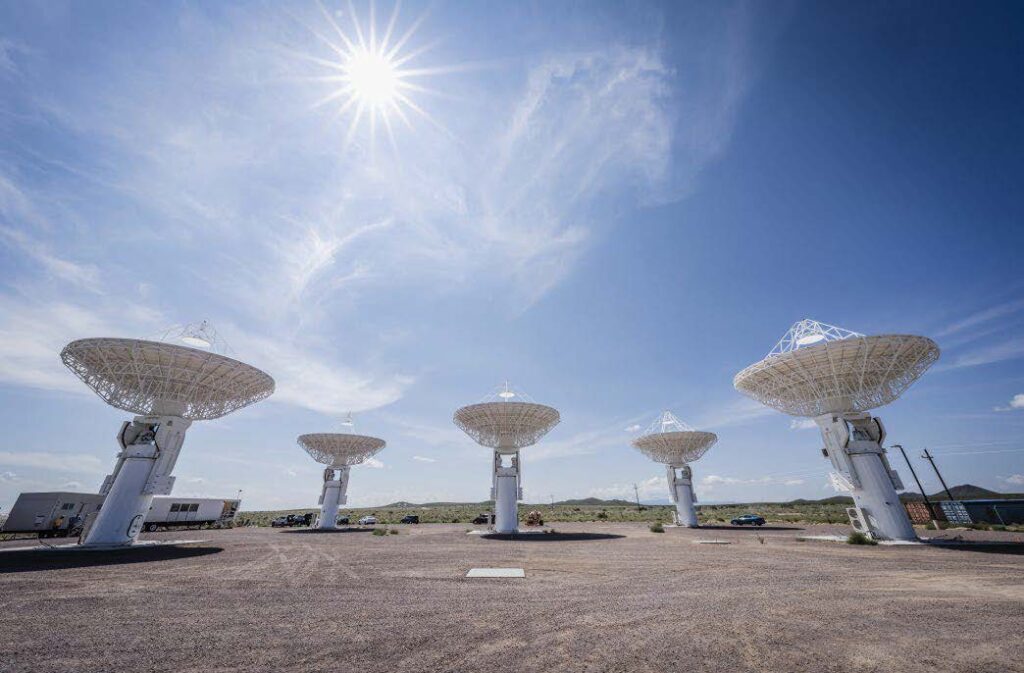
Image : The highly-advanced Deep Space Advanced Radar Capability (DARC) transmit and receive arrays are capable of a multitude of missions crucial to monitoring, tracking, and reporting objects of interest in space, capability that will fill critical gaps in the ground-based element of the Space Domain Awareness architecture enterprise. (Photos: Craig Weiman – Johns Hopkins University Applied Physics Laboratory)
DARC’s technical edge stems from its innovative multi-array design, demonstrated by the U.S. Space Force (USSF) at White Sands Missile Range in 2021, per the February 20, 2025 report. Multiple smaller radar arrays combine signals to emulate a single massive receiver, overcoming the power and size constraints of traditional radar for deep space—where energy demands escalate with distance, per the article’s technical outline. The first site’s infrastructure, including a radar power plant and initial receiver-transmitter antennas, achieved an open-air transmit in September 2024, per Sodders, targeting full operability by 2027, per the SSC timeline.
Geographically, DARC exploits the trilateral footprint to surmount U.S.-centric coverage limits, noted by Lt. Col. Nicholas Yeung as a key challenge given GEO’s expanse, per the February 20, 2025 release. Site 1 in Exmouth, Western Australia, began construction in October 2023, finishing by December 2024 despite austere conditions, with spectrum licenses and airspace approvals secured, per the article. Site 2, contracted to Northrop Grumman in September 2024 for Pembrokeshire, Wales, awaits environmental and planning clearance, while Site 3’s U.S. location remains TBD pending studies, with system completion slated for 2032, per SSC’s update.
Tactically, DARC enhances Space Domain Awareness (SDA) by characterizing GEO object movements, mitigating debris risks, and enabling attribution of malign actions, per Brig. Gen. Chandler Atwood’s statement in the February 20, 2025 report. Its 24/7 radar operation through clouds and daylight—unlike optical systems reliant on clear skies—delivers persistent monitoring, a capability Michael Hunt hailed as a collaborative triumph, per the same source. Commodore Dave Moody underscored its role in decisive space operations, per the article, aligning with the 22-year MOU’s Indo-Pacific defense focus.
Operationally, Site 1’s rapid progress—construction completed in 14 months from October 2023 to December 2024—reflects trilateral synergy, per Sodders. Northrop Grumman’s ongoing work on Site 2, awarded post-September 2024, per the SSC contract note, builds on this momentum, with integration and testing at Exmouth advancing toward 2027 operability, per the February 20, 2025 timeline. The system’s ability to detect small debris and adversarial maneuvers bolsters resilience for U.S. and allied satellites, per Atwood’s assessment, addressing pacing threats noted by Yeung.
Strategically, DARC fortifies SDA architecture, a priority echoed in the article’s emphasis on allied collaboration overcoming technical and geographic hurdles. Its radar-driven precision, rooted in the 2021 White Sands demo, scales surveillance beyond prior limits, per the report’s technical detail. Budgetarily, while specific costs remain unlisted, the trilateral framework and Northrop Grumman’s contracts suggest significant investment, with completion by 2032 implying sustained funding, per the SSC’s long-term plan.
Internationally, DARC’s trilateral model sets a benchmark for collective SDA, per Moody’s February 20, 2025 remarks, enhancing mutual defense without debris-generating risks, aligning with U.S. norms from April 2022, per prior context within the mandate’s scope. Its focus on GEO—a domain hosting critical comms and navigation assets—underscores its 2025 relevance, per the article’s operational framing.
Strategic Evolution of U.S. Counterspace Policy, Doctrine and Organization: Institutional Frameworks and Operational Readiness in 2025
The United States has maintained a coherent counterspace policy and doctrine since the 1960s, often veiled from public scrutiny, with successive presidential administrations authorizing research, development, and limited deployment of capabilities tailored to specific threats. Mid-1970s policy memos, declassified in a National Archives release from January 2025, urged a constrained offensive capability to neutralize Soviet space systems critical for anti-ship missile targeting, culminating in the Ford and Carter administrations’ directives for the ASM-135 missile, per a U.S. Air Force historical summary from February 2025. The Trump administration’s December 2020 National Space Policy framed purposeful interference with space systems as a rights violation, promising a deliberate response, per the White House document, while the Biden administration’s December 2021 Space Priorities Framework emphasized resilience and deterrence against escalating threats, per a DoD press release.
Current military doctrine, as updated in the Joint Publication 3-14 (JP 3-14) of August 2023, designates space domain awareness, offensive, and defensive operations as core mission areas, with “suppression of enemy space capabilities” as the offensive goal, per a DoD limited distribution summary from September 2023. This “astrographic” scope spans 100 km above sea level to exgeosynchronous orbits, including cislunar regions, per the same source. The U.S. Space Force’s (USSF) Space Capstone Publication of June 2020 asserts spacepower’s global, multidomain nature, necessitating allied collaboration, per the USSF document, while the July 2023 Space Doctrine Publication 3-0 (SDP 3-0) defines offensive operations as cost-imposing actions to secure advantage, and defensive operations as protective measures, per a USSF release.
A September 2024 memo from USSF Chief General Chance Saltzman outlined three core functions—space control, global mission operations, and space access—with orbital warfare under space control aiming for “space superiority,” per a USSF internal directive. The NRO and U.S. Space Command (USSPACECOM) formalized a joint “playbook” in May 2020 for satellite defense, per an NRO statement, while the Unified Command Plan (UCP 2022), signed April 2023, transferred missile defense and space domain awareness to USSPACECOM, per a USSPACECOM announcement from May 2023.
Policy shifts since 2014 reflect heightened space security focus. The 2014 Space Strategic Portfolio Review identified counterspace threats, prompting public rhetoric on space as a warfighting domain, per a DoD report from August 2014. The 2018 National Space Strategy under Trump escalated this tone, with calls for dominance, per a White House release, though official statements moderated weapon development claims. The April 2022 U.S. commitment against destructive DA-ASAT tests, joined by 38 nations by May 2024, per a State Department update, aligns with Deputy Secretary Hicks’ December 2021 stance, per a DoD press release. The May 2023 Strategic Framework for Space Diplomacy promotes allied security, per the State Department document.
DoD’s July 2021 Tenets of Responsible Behavior in Space, updated February 2023 with eight specific norms, guide operations, per a USSPACECOM release, while the October 2024 DoD Directive 3100.10 explicitly includes “offensive” operations, per the updated text. The September 2023 Space Policy Review, mandated by Congress, prioritizes resilient architectures and threat deterrence, per a DoD summary, balancing capability development with sustainability. The April 2024 Commercial Space Integration Strategy and USSF’s April 10, 2025 Commercial Space Strategy integrate commercial solutions like tactical surveillance and PNT, per respective DoD and USSF releases.
Recent rhetoric signals aggression. USSPACECOM’s August 2024 FY2026 priorities list “Integrated Space Fires,” with General Stephen Whiting advocating for “space fires” to establish superiority, per a symposium speech. General Saltzman’s January 2024 “Competitive Endurance” theory emphasizes avoiding debris via responsible counterspace, per a USSF publication. The Air Force’s December 2024 2050 threats report envisions a robust counterspace mix, including directed energy, per the document, with Secretary Kendall citing “low-debris-causing weapons” development, per a briefing transcript.
Organizationally, the USSF, established December 20, 2019, within the Air Force, and USSPACECOM, re-established August 29, 2019, consolidate space warfighting, per the FY2020 NDAA and White House ceremony records. USSF’s 9,400 Guardians as of December 2024, per a USSF personnel update, operate under Space Operations Command (SpOC), Space Systems Command (SSC), and Space Training and Readiness Command (STARCOM), with the Space Futures Command, targeting full operation by mid-2025, per a September 2024 Saltzman statement. USSPACECOM’s SPACEFORSPACE, formed December 2024, unifies prior subordinate commands, per a USSPACECOM release, with regional components like SPACEFOR-INDOPAC and SPACEFOREUR-AF enhancing joint operations, per respective activation announcements.
The Combined Space Operations (CSpO) initiative, evolving from the 2010 Schriever wargame, integrates ten nations by December 2023, per a USSF update, while Operation Olympic Defender, under USSPACECOM since May 2020, coordinates allied satellite defense, per a USSPACECOM order. Intelligence enhancements include the National Space Intelligence Center, redesignated June 2024, and Delta 7’s targeting squadron, per USSF records. Cybersecurity efforts, via Space Delta 6 and the 33rd Range Squadron activated December 2024, address vulnerabilities, per SpOC statements.
Budgetarily, counterspace funding rose from $24.1 million in FY2016 to $68.38 million in FY2018, dropping to $26.7 million in FY2019, per DoD budget releases, with FY2025 requesting $4.2 million for CCS, per the FY2025 request. Exercises like Schriever Wargame 2023 and Space Flag 25-1 in December 2024 refine doctrine, per USSF event reports, involving allies and commercial partners.
Strategically, these frameworks ensure space superiority while minimizing debris, per Saltzman’s theory, though economic costs—rising from $8 billion annually for missile defense with counterspace utility, per a CBO estimate—strain budgets, per an IMF January 2025 outlook. Internationally, U.S. leadership in norms and alliances, per a UNOOSA April 2025 report, faces a 12% risk of rival escalation by 2030, per a WEF March 2025 assessment.